Whole-body Expansion Microscopy
Seeing is believing.
In brief
Interpreting WHOLISTIC data critically requires good anatomical knowledge of the larval zebrafish to infer cell-type identity, yet available resources on larval zebrafish internal anatomy are scarce. We found that we were consistently limited by a lack of access to high-resolution anatomical and cell-type references, especially for the viscera. While there are many high-quality references and resources for the central nervous system of larval zebrafish1,2,3, body-wide datasets have limited resolution or have used X-ray4 and do not include molecular information. Changes in the refractive index in the viscera make the acquisition of high-quality data a challenge even after tissue clearing. Expansion microscopy (ExM) offers a ready solution to probing for proteins and RNA in thick tissues by rendering tissue nearly perfectly index matched to water. However, most existing ExM protocols have been optimized for brain tissue, and methods that target whole fish either perform best in early-stage embryos5 or require weeks-long, aggressive chemical anchoring and enzymatic digestion steps to digest the heterogenous structures including cartilage that appear in later-stage larvae6. We developed an enzyme-free, robust, and rapid method for whole-body expansion that performs well up to at least 21-dpf larvae. Briefly, we reasoned that high-temperature (100ºC) chemical hydrolysis would soften tissue more uniformly and controllably than enzymatic proteolysis; embedding in a medium-density gel with low protein-gel anchoring prevented cracks from forming prior to digestion; repeated embedding with modest $\sim$1.5x expansion factors in each round produced more reliable tear-free expansion while any tears that do form in tough tissue structures such as fine cartilage tend not to propagate to surrounding soft tissue. The lack of aggressive enzymatic proteolytic digestion in the protocol means that signal preservation from pre-gelation immunofluorescence (IF) is maximized throughout the protocol. Furthermore, while RNA is not persevered at 100ºC, using the developed ideas above, re-introducing relatively short proteinase K digestion steps instead of 100ºC hydrolysis allows acceptable expansion and clearing performance to be achieved while preserving RNA for later probing in the expanded gel.
Population-level cellular dynamics
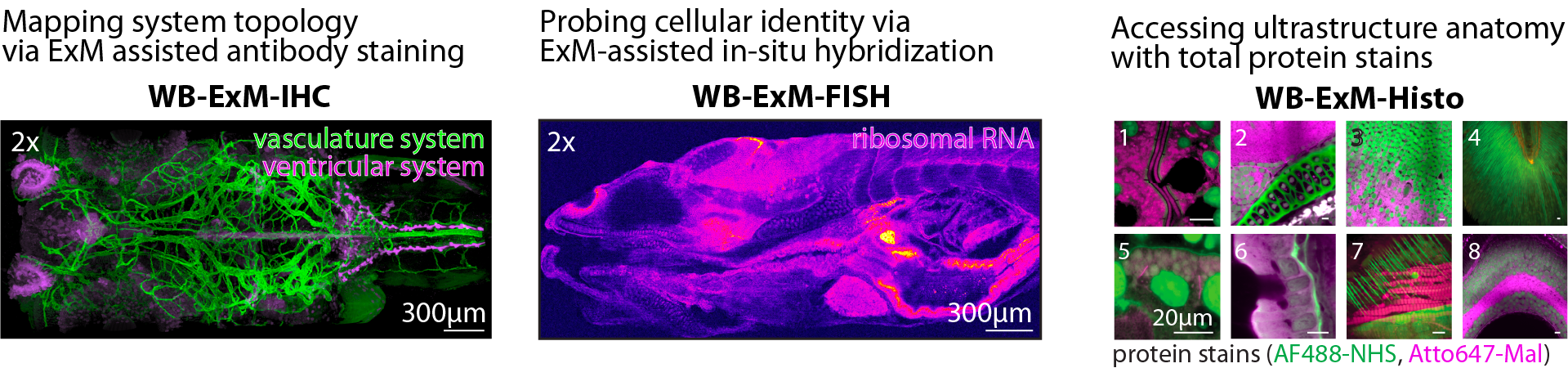
What is Expansion Microscopy?
Expansion microscopy (ExM) is a form of super-resolution microscopy. Unlike standard super-resolution methods, which either focus on altering the hardware or software, ExM focuses on altering the object to be imaged itself, making it physically larger7. ExM considers native tissue as a physical network of biomolecules. Throughout this biological network, an artificial acrylamide-based polymer network can be interlaced. This artificial network can be made to molecularly bind to the biological network via the use of NHS (hydroxysuccinimide ester) chemistry: an NHS moiety is added to the polymer monomer (together called AcX for short). This NHS group can then undertake a nucleophilic attack to amine groups found within the tissue, for instance on lysines, a common amino acid present in essentially all proteins. To be able to move endogenous proteins apart, one needs to first reduce the number of crosslinks that keep the tissue together. Endogenous bonds can be disrupted by detergents, denatured, or cleaved. This reduces the number of forces that hold endogenous proteins together. Finally, by reducing the salt concentration of the medium, the artificial network can be made to swell. The swellable nature of the gel comes from the fact that the acrylate molecules are part of the acrylamide backbone. These charged components naturally want to repel each other; salt masks these charges, thus making the gel more compact at high salt levels. As the natural network of proteins is bound to the acrylamide gel, when the gel swells, the natural network of proteins expands with it. This results in molecules of interest being further apart and thus more readily resolvable. Lipids are typically dissolved away because they are not bound to the gel and the density of proteins left is much reduced, which together results in fewer refractive interfaces, lowering the spatial variation in refractive index, and leading to a cleared, optically accessible sample. The interaction between the properties of biological and artificial networks and how they are assembled determines the quality of the outcome, including the expansion, homogeneity, and retention of molecules. Most of the work involves navigating this design space with now many variations of original protocol published having been optimized for specific samples and applications such as tissue thickness or expansion factor8,9.
Why Expand?
Interpreting Whole Body Imaging (WBI) data relies heavily on having good anatomical knowledge of larval zebrafish anatomy. Indeed, WBI trades-off cell identifiabilty for tissue coverage: by labeling all cells one loses ground-truth knowledge of cell-type identity. This trade-off, which could have been very steep, in practice was found to be modest as a surprisingly large amount of tissue types can be distinguished by eye. This however requires a good grasp of the 3D organization of tissues within the animal, and the richer ones prior about tissue and cellular morphology, the more inferences one can make when analyzing WBI data. As such, analyzing functional data became inseparably intertwined with tissue type identification, the latter often becoming a limiting step, and calling for the gain of more granular anatomical knowledge of the fish.
Despite the larval zebrafish being an established model organism, comprehensive anatomical information for tissues other than the brain could be difficult to come by: existing high-resolution zebrafish atlases focus on the brain and central nervous system (e.g. MPI1, Harvard2, NIH3 and body-wide datasets have limited resolution or have used X-ray (Penn State4 and do not include molecular information. The reason for the lack of published high-quality anatomical data, especially for inner organs, soon became clear as we attempted to collect our own anatomical reference data: despite the claims that larval zebrafish are transparent, the accumulation of changes in refractive index across the sample causes tissue scattering, which only worsens after fixation, making it difficult to collect clean data. Clearing protocols only made partial dents in this problem, and penetration of antibodies across the tissue was also an issue; indeed, many protocols ask users to remove the skin of the sample (e.g.:10 which comes at the risk of causing tissue disruption and warping. As such, this was a methodological problem. This chapter describes how we addressed this gap by leveraging advances in Expansion Microscopy and developing a protocol enabling the acquisition of high-resolution whole-body anatomical and molecular data, crucial to being able to interpret and make the most of WBI data.
Expansion microscopy (ExM) offers a ready solution to accessing deep tissue and probing for proteins and RNA. However, most existing ExM protocols have been optimized for brain tissue, a highly homogeneous tissue. The diversity of tissue types within a whole larva, compared to the brain alone, makes isotropic expansion more difficult to achieve. The challenges arise in part because the cross-link density between the sample and gel depends on the local tissue ultrastructure, and variations in this lead to inhomogeneous expansion and, worse, to tearing. To overcome this, strong proteolysis can be induced to break up protein networks (e.g.: using proteinase K), but at the cost of signal retention. Navigating the landscape of dependencies between recipe parameters is challenging, as the number of parameters is vast and interactions complex, and there is no high-throughput way to measure expansion uniformity and signal retention simultaneously. Furthermore, as the animal grows, tissue heterogeneity only amplifies with, for instance, the rapid expansion of cartilage, which begins around 6 days post-fertilization (d.p.f). Our initial attempts to apply existing protocols, optimized for larval brain tissue, to the entire fish led to large tears and deformations. \bigskip
Prior attempts in the literature
Two whole-fish ExM protocols have been published; the first uses the original low monomer ExM recipe and strong digestion with extensive proteinase K incubations, which comes at the cost of protein loss, and the article lacks scrutinizeable IHC results (only one supplementary figure is present for a 3 d.p.f. fish, showing a superficial structure)6. This group presents data showing good whole-body morphology; however, the oldest sample shown in detail is 6 d.p.f. (younger than that typically imaged during WBI). The protocol takes about 2 weeks. The second published whole-fish ExM protocol shows post-expansion IHC results5. It overcomes the problem of expanding heterogeneous tissue by increasing the concentration of acrylamide (i.e., making a tougher gel); however, the protocol is only applied to samples of up to 5 d.p.f., which is the age just before when we begin to see large tears with the onset of cartilage growth. The protocol also takes about 2 weeks.
In need of a protocol compatible with both maximizing retention of pre-expansion IHC signals and using older ages of fish, mentored by Paul Tillberg and helped by Amy Hu and Mark Eddison, we developed a new whole-body ExM protocol, achieving both by adopting a new strategy: instead of attempting to make a single tough gel, we decreased the acrylamide concentration and used a multi-embedding approach combined with very high temperature (100 °C) disruption that enables uniform expansion without protease treatment, nor concomitant protein loss. The interlacing of polymers enables a gentler and gradual expansion. The resulting method takes a total of 1 week from fixation to imaging, reaches up to 4x expansion, and also works with older samples (21 d.p.f. zebrafish and mature Danionella. Finally, to facilitate data analysis, we also optimized sample mounting with the inclusion of an agarose pre-embedding step and enlarging the chamber to ensure consistent sample orientation and avoid edge artifacts. We provide a detailed user-friendly protocol to facilitate method uptake.
Protocol
All protocols can be found in detail on protocols.io.
WB-ExM-IHC: Detailed Protocol
WB-ExM-FISH: Detailed Protocol
WB-ExM Protocol
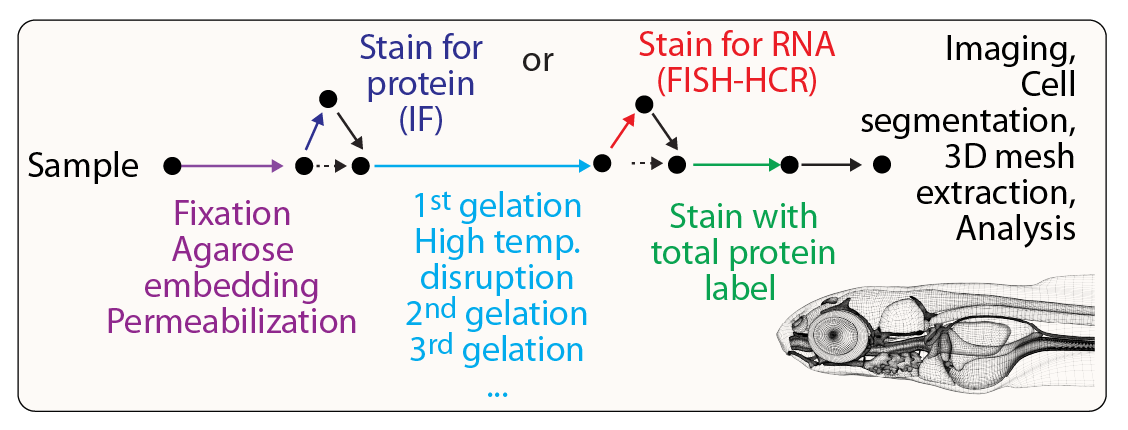
Outline of WB-ExM protocol. Following fixation, embedding and permeablization, samples undergo repeated rounds of gel-embedding allowing for progressive reinforcement and expansion of the specimen.
If you want to try out the protocol, don't hesitate to reach out if you have any questions! Contact us via email.
References
- 1. A Cellular-Resolution Atlas of the Larval Zebrafish Brain, Neuron, 2019, Kunst, Michael and Laurell, Eva and Mokayes, Nouwar and Kramer, Anna and Kubo, Fumi and Fernandes, Ant{\'{o}}nio M. and F{\"{o}}rster, Dominique and {Dal Maschio}, Marco and Baier, Herwig
- 2. Whole-brain activity mapping onto a zebrafish brain atlas, Nature Methods, 2015, Randlett, Owen and Wee, Caroline L. and Naumann, Eva A. and Nnaemeka, Onyeka and Schoppik, David and Fitzgerald, James E. and Portugues, Ruben and Lacoste, Alix M.B. and Riegler, Clemens and Engert, Florian and Schier, Alexander F.
- 3. Brain-wide cellular resolution imaging of cre transgenic zebrafish lines for functional circuit-mapping, eLife, 2019, Tabor, Kathryn M. and Marquart, Gregory D. and Hurt, Christopher and Smith, Trevor S. and Geoca, Alexandra K. and Bhandiwad, Ashwin A. and Subedi, Abhignya and Sinclair, Jennifer L. and Rose, Hannah M. and Polys, Nicholas F. and Burgess, Harold A.
- 4. Computational 3d histological phenotyping of whole zebrafish by x-ray histotomography, eLife, 2019, Ding, Yifu and Vanselow, Daniel J. and Yakovlev, Maksim A. and Katz, Spencer R. and Lin, Alex Y. and Clark, Darin P. and Vargas, Phillip and Xin, Xuying and Copper, Jean E. and Canfield, Victor A. and Ang, Khai C. and Wang, Yuxin and Xiao, Xianghui and Carlo, Francesco De and Rossum, Damian B.Van and Riviere, Patrick La and Cheng, Keith C.
- 5. TissUExM enables quantitative ultrastructural analysis in whole vertebrate embryos by expansion microscopy, Cell Reports Methods, 2022, Steib, Emmanuelle and Tetley, Rob and Laine, Romain F. and Norris, Dominic P. and Mao, Yanlan and Vermot, Julien
- 6. Nanoscale resolution imaging of the whole mouse embryos and larval zebrafish using expansion microscopy, bioRxiv, 2022, Sim, Jueun and Park, Chan E and Cho, In and Min, Kyeongbae and Eom, Minho and Han, Seungjae and Jeon, Hyungju and Cho, Hyun-Ju and Cho, Eun-Seo and Kumar, Ajeet and Chong, Yosep and Kang, Jeong Seuk and Piatkevich, Kiryl D and Jung, Erica E and Kang, Du-Seock and Kwon, Seok-Kyu and Kim, Jinhyun and Yoon, Ki-Jun and Lee, Jeong-Soo and Boyden, Edward S and Yoon, Young-Gyu and Chang, Jae-Byum and Paulson, John A
- 7. Expansion microscopy, Science, 2015, Chen, Fei and Tillberg, Paul W and Boyden, Edward S
- 8. EASI-FISH for thick tissue defines lateral hypothalamus spatio-molecular organization, Cell, 2021, Wang, Yuhan and Eddison, Mark and Fleishman, Greg and Weigert, Martin and Xu, Shengjin and Wang, Tim and Rokicki, Konrad and Goina, Cristian and Henry, Fredrick E. and Lemire, Andrew L. and Schmidt, Uwe and Yang, Hui and Svoboda, Karel and Myers, Eugene W. and Saalfeld, Stephan and Korff, Wyatt and Sternson, Scott M. and Tillberg, Paul W.
- 9. Unclearing Microscopy, bioRxiv, 2022, M'Saad, Ons and Shribak, Michael and Bewersdorf, Joerg
- 10. A new mode of pancreatic islet innervation revealed by live imaging in zebrafish, eLife, 2018, Yang, Yu Hsuan Carol and Kawakami, Koichi and Stainier, Didier Y.R.