Drug screens
Beyond enabling progress in fundamental biology, WBI enables drug screens in disease models that allow for tracking the effects at multiple spatial scales from cells to the whole body and at temporal scales from seconds to days. The majority of drug-screening workflows depend on observing a subset of drug-induced phenomenology such as the molecular binding properties1, the effects on individual cells or subset of tissues2 or influence on behavior3,4. WBI offers a complementary lens through which to observe the impact of drugs and genetic interventions on the entire body, enabling the identification of unanticipated off-target consequences in organ systems not under direct study, as well as the discovery of unexpected benefits in tissues not previously considered. It would also reveal outcomes arising from system-wide interactions that could be missed when studying cells, tissues, or organs individually, rather than collectively within their native whole-body environment. Ultimately, combining WBI with fictive-swimming virtual-reality systems5 will enable research at the intersection of whole-body cellular physiology, systems neuroscience, and behavior, shedding light on the interactions between interoception and exteroception, as well as the interplay and feedback between cognition and visceral state in driving behavior and physiology.
Example: WBI identifies new cellular response properties to Ketamine
As a demonstration of using WBI for drug screening, we performed a WBI screen for cells that respond to ketamine, a dissociative anesthetic. Quantifying the strongest responding cells by ranking Fnrom increases, we identified a functional compartment that aligns with the outer perimeter of the brain, likely corresponding to the brain meninges, which is responsive to ketamine. While the effects of ketamine on neurons and glia are documented6, the role of the meninges is less studied; meninges have not yet been functionally imaged in any species in vivo7 and remain largely uncharacterized in zebrafish. With no existing selective driver line8, they are difficult to segment based on anatomy due to the thinness of the tissue. This highlights how WBI activity analysis is able to delineate functional compartments that are otherwise challenging to label – i.e., identify, within the many cells in the body, small groups of cells with specific dynamics that would otherwise remain undetected.
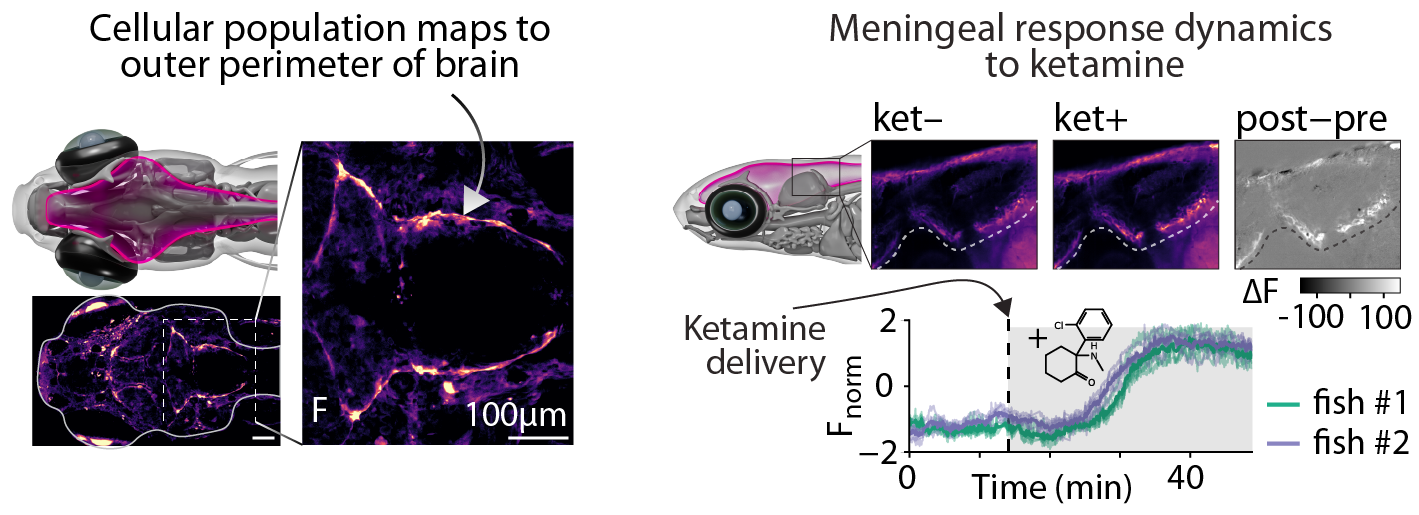
Meningeal response to ketamine exposure. Animals were exposed to ketamine following a 25 min period of baseline imaging. Top) Sagittal section showing pre- and post-exposure to ketamine; Bottom) activity traces from meningeal regions.
References
- 1. An artificial intelligence accelerated virtual screening platform for drug discovery, Nature Communications, 2024, Zhou, Guangfeng and Rusnac, Domnita-Valeria and Park, Hahnbeom and Canzani, Daniele and Nguyen, Hai Minh and Stewart, Lance and Bush, Matthew F. and Nguyen, Phuong Tran and Wulff, Heike and Yarov-Yarovoy, Vladimir and Zheng, Ning and DiMaio, Frank
- 2. Therapeutic landscape of metabolic dysfunction-associated steatohepatitis ({MASH}), Nature Reviews Drug Discovery, 2024, Do, Albert and Zahrawi, Frhaan and Mehal, Wajahat Z.
- 3. Deep phenotypic profiling of neuroactive drugs in larval zebrafish, Nature Communications, 2024, Gendelev, Leo and Taylor, Jack and Myers-Turnbull, Douglas and Chen, Steven and McCarroll, Matthew N. and Arkin, Michelle R. and Kokel, David and Keiser, Michael J.
- 4. Zebrafish disease models in drug discovery: from preclinical modelling to clinical trials, Nature Reviews Drug Discovery, 2021, Patton, E. Elizabeth and Zon, Leonard I. and Langenau, David M.
- 5. Two-photon calcium imaging during fictive navigation in virtual environments, Frontiers in Neural Circuits, 2013, Ahrens, Misha B. and Huang, Kuo Hua and Narayan, Sujatha and Mensh, Brett D. and Engert, Florian
- 6. Ketamine induces plasticity in a norepinephrine-astroglial circuit to promote behavioral perseverance, Neuron, 2024, Duque, Marc and Chen, Alex B. and Hsu, Eric and Narayan, Sujatha and Rymbek, Altyn and Begum, Shahinoor and Saher, Gesine and Cohen, Adam E. and Olson, David E. and Li, Yulong and Prober, David A. and Bergles, Dwight E. and Fishman, Mark C. and Engert, Florian and Ahrens, Misha B.
- 7. Meningeal cells can communicate with astrocytes by calcium signaling, Annals of neurology, 2000, Grafstein, Bernice and Liu, Shujun and Cotrina, Maria Luisa and Goldman, Steven A and Nedergaard, Maiken
- 8. Molecular and Cellular Analysis of the Repair of Zebrafish Optic Tectum Meninges following Laser Injury, Cells, 2022, Banerjee, Payel and Joly, Paul and Jouneau, Luc and Jaszczyszyn, Yan and Bourge, Micka{\"{e}}l and Affaticati, Pierre and Levraud, Jean Pierre and Boudinot, Pierre and Joly, Jean St{\'{e}}phane